Kate E Galloway
synthetic biology. molecular systems biology. cell fate circuits.
DRIVING CELLULAR REPROGRAMMING
Cellular reprogramming holds great potential to generate rare, inaccessible cell types for disease-modeling. However, conversion has been limited by inefficient reprogramming requiring large-scale efforts to generate only a few hundred cells. Moreover, the central mechanistic rules for direct lineage conversion remained obscure. Today, as a direct result of my work to improve the reprogramming process, I can robustly generate thousands of cells with signatures of enhanced maturity.
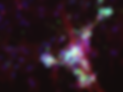
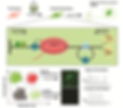
CELLULAR HARDWARE LIMITS REPROGRAMMING
In addition to improving the reprogramming process, my work uncovers a previously unrecognized explanation for why only rare cells undergo transcription factor-mediated reprogramming successfully. I found that cellular reprogramming is limited to a small population of cells equipped to process the dual, competing demands of hyperproliferation and hypertranscription. High rates of transcription and replication accelerate the rate of DNA tangling (e.g. supercoiling). Only cells equipped with high expression of topoisomerases, enzymes that relax DNA supercoils, are capable of mediating the massive genomic and transcriptional realignment to convert from one state to another.
TANGLED WIRES
My findings suggest that topological stress profoundly impacts the function of gene networks (e.g. native or synthetic circuits). By more precisely defining the impact of topological stress on gene circuits, I will elucidate principles for buffering (or alternatively, harnessing) topological stress to enhance the performance and capabilities of genome-integrated synthetic circuits.
​
Relevant Publications:
​
1. Babos, K.N.*, Galloway, K.E.*, et al. Balancing dynamic tradeoffs to drive cellular reprogramming.
​
2. Galloway, KE and Ichida, JI. Stem Cells, Tissue Engineering and Regenerative Medicine. 2015).
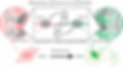
IDENTIFYING PATHWAY REGULATORS
By identifying pathway regulators of MAPK signaling in yeast (S. cerevisiae), I constructed synthetic gene circuits and used these regulators to develop a class of genetic control systems called molecular network diverters.

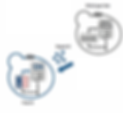
DYNAMICALLY PROGRAMMING CELL FATE
By interfacing these molecular network diverters with the native MAPK signaling pathway, I could temporally and spatially control cellular decision-making events. My model system suggested how similar pathways in mammalian cells could be controlled to regulate cell fate.
PRINCIPLES OF INTEGRATING CIRCUITS
INTO NATIVE PATHWAYS
Beyond controlling cell fate, the design and construction of molecular network diverters allowed me to explore fundamental principles of integrating native and synthetic circuitry. For example, my work highlighted that integrated negative regulators can buffer a system against noise amplification mediated through positive-feedback loops by providing a resistance to amplification. In the future, I will use my expertise in constructing synthetic circuitry to illuminate paradigms in biological control and apply those principles to enhance cellular programming.
​
Relevant Publications:
1. Galloway, K.E. et al. Science 2013.
2. Galloway, K.E. and Franco, E. Computational Methods in Synthetic Biology, 2015).
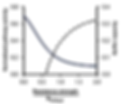